SERUM NICOTINAMIDE ADENINE DINUCLEOTIDE LEVELS THROUGH DISEASE COURSE IN MULTIPLE SCLEROSIS
Nady Braidya,1, Chai K. Limb,1, Ross Grantc,d, Bruce J. Brewe,Gilles J. Guilleminb,n
aCentre for Healthy Brain Ageing, School of Psychiatry, University of New South Wales, Sydney, Australia bNeuropharmacology group, MND and Neurodegenerative diseases Research Centre, Macquarie University, Balaclava Road, North Ryde, Sydney, NSW 2109, Australia cAustralasian Research Institute, Sydney Adventist Hospital, Wahroonga, Sydney, Australia dDepartment of Pharmacology, School of Medical Sciences, University of New South Wales, Sydney, Australia eDepartment of Neurology, St Vincent’s Hospital, Sydney, Australia
Brain Research ( 2 0 1 3 ) 2 6 7 – 2 7 2
ABSTRACT
The levels of the essential pyridine nucleotide, NADþ and its reduced form NADH have not been documented in MS patients. We aimed to investigate NADþ and NADH levels in serum in patients with different disease stages and forms of MS. NADþ and NADH levels were measured in the serum from 209 patients with relapsing remitting MS (RRMS), 136 with secondary progressive MS (SPMS), 51 with primary progressive MS (PPMS), and 99 healthy controls. All patients were in a clinically stable phase. Serum NADþ levels declined by at least 50% in patients with MS compared to controls (17.973.2 μg/ml; p ¼ 0.0012). Within the MS sub-groups NADþ levels were higher in RRMS (9.972.9 μg/ml; p ¼ 0.001) compared to PPMS (6.372.1 μg/ml; p ¼ 0.003) and SPMS (7.872.0 μg/ml; p ¼ 0.005). A two-fold increase in NADH levels (p ¼ 0.002) and at least three-fold reduction in the NADþ/NADH ratio (p ¼ 0.009) were observed in MS patients compared to controls. Serum NADþ and NADH levels are may be associated with disease progression in MS. Given the importance of NADþ in the maintenance of normal cellular function, it is likely that this molecule is of therapeutic relevance in MS.
INTRODUCTION
Nicotinamide adenine dinucleotide (NADþ) and reduced nicotinamide adenine dinucleotide (NADH) are ubiquitous pyridine nucleotides which are well known to participate in oxidation–reduction reactions during ATP production (Berger et al., 2005). NADþ also serves as a cofactor for NAD-dependent glycohydrolases (CD38) involved in intracel- lular calcium regulation (Berger et al., 2004). NADþ serves as an important secondary messenger signaling molecule indu- cing the continuous release of intracellular calcium to med- iate lymphocyte chemotaxis (Partida-Sanchez et al., 2007) or microglia activation (Lior et al., 2008). Recently, NADþ has also been shown to act as the sole substrate for the DNA nick sensor poly(ADP-ribose) polymerase-1 (PARP-1), and the class III protein lysine deacetylases, the sirtuins (silent information regulators of gene transcription).
On the contrary, NADH is a coenzyme which can stimulate energy production by replenishing depleted cellular stores of ATP. However, when the re-oxidation of NADH is impaired due to reduced complex I activity, the NADH/NADþ ratio increases, thus reducing the activity of several NADþ dependent dehy- drogenase enzymes. Moreover, NADH is also able to promote the formation of H2O2 in the presence of iron via Fenton chemistry leading to further oxidative stress formation and NADþ depletion (Tretter and Adam-Vizi, 2004). The NADþ/ NADH ratio plays an omnipresent role in regulating the intracellular redox status, and therefore represents a function of the metabolic state (Massudi et al., 2012a; Massudi et al., 2012b). Given the major function of these two nucleotides in maintaining normal cellular homeostasis during inflamma- tion, further studies into the biological roles of NADþ and NADH may increase our understanding for the potential role of NADþ related therapies in MS (Massudi et al., 2012b).
During chronic CNS inflammation, oxidative stress may potentially play a critical role in the demyelination and neuro- degeneration observed in MS (Koch et al., 2006). NADþ/NADH levels are altered by Th-1 derived cytokines such as IFN-γ, which enhance free radical generation during inflammation, and increase PARP activity and therefore NADþ catabolism (Grant and Kapoor, 2003; Grant and Kapoor, 1998; Grant et al., 2000). Persistent activation of PARP, has been shown to stimu- late NADþ depletion in human neuronal cells (Braidy et al., 2009; Wang et al., 2003). Reduced NADþ levels appear to result in a loss of cellular function and metabolism culminating in cell death [16]. Retention of intracellular NADþ/NADH pools may therefore facilitate PARP dependent nuclear repair and ATP production following an oxidative insult in vulnerable neurons in MS (Penberthy and Tsunoda, 2009). There is also controver- sial evidence that lowered NADþ levels may be associated with systemic effects such as fatigue, a common complaint as MS progresses (Lassmann, 2008).
To our knowledge, there is no information regarding the role of NADþ and NADH in MS. As these pyridine nucleotides play critical roles in maintaining cell survival via several mechanisms, it is important to determine changes in the levels of NADþ and NADH in the pathogenesis of MS. We report here on a cross-sectional study of changes in serum NADþ/NADH levels as measured by a well-established spectro- photometric technique in various sub-groups of MS patients.
RESULTS
The data presented in Fig. 1 and Table 1 show that the serum NADþ levels were significantly lower in patients with MS compared to controls (p ¼ 0.0012) when corrected for age and gender. Higher levels of NADH (Fig.2, p ¼ 0.002) and a lower NADþ/NADH ratio (Fig. 3, p ¼ 0.009) were observed in MS patients compared to controls. Although higher NADþ (including NADþ and NADH) levels were observed in males compared with females in the control group and in patients with MS, this did not reach statistical significance (Table 2).
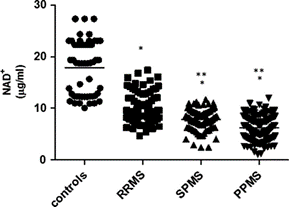
Fig. 1 – Serum NADþ levels in different MS disease course and in controls. Note:- PPMS indicates primary progressive multiple sclerosis; SPMS, secondary progressive multiple sclerosis; RRMS, relapse-remitting multiple sclerosis.npo0.05 Compared to serum from healthy controls.nnpo0.05 Compared to serum from RRMS patients.
NADþ levels were significantly different between the MS subgroups (P ¼ 0.0012), with higher levels in RRMS (p ¼ 0.001) compared to PPMS (p ¼ 0.003) and SPMS (p ¼ 0.005) when corrected for age and gender. Serum NADþ levels were not statistically significant between PPMS and SPMS (p40.05). NADH levels and NADþ/NADH ratio were not significantly different between the MS subgroups (P40.05).
DISCUSSION
The present study demonstrates that NADþ and NADH levels are significantly altered in MS. While NADH levels and NADþ/ NADH ratio were not significantly different between MS sub- groups, NADþ levels were markedly lower in PPMS and SPMS compared to RRMS. Altered NADþ metabolism has been observed in progressive neuronal cell death both in vivo and in vitro (Arraki et al., 2004). Below we will discuss how our findings subjectively fit into the current understanding of MS pathogenesis.
One of the major contributing factors towards NADþ depletion is chronic oxidative stress leading to reduce cellular viability. Oxidative stress can be mediated by increased reactive oxygen species (ROS) and subsequently worsen when the cellular capability of detoxifying ROS is compromised. ROS produced in MS and experimental autoimmune encephalomyelitis (EAE) – the most common animal model of MS – are thought to be released from activated inflammatory cells and are directly involved in demyelination and axonal damage (Gilgun-Sherki et al., 2004). Increased oxidative stress damages DNA and activates PARP-1, which utilizes NADþ as the substrate (Szabo, 2006).
Under mild to moderate ROS mediated DNA damage, activation of PARP-1 can lead to DNA repair and restoration of normal cellular function. Similarly, up regulation of PARP-1 activity has been observed in activated glial cells surrounding demyelinated EAE plaques (Kauppinen et al., 2005). Additionally, Diestel et al. (2003) showed that the levels of 7-ketocholesterol, an important lipid breakdown product, is increased in the brain and cerebrospinal fluid (CSF) of MS patients and in EAE mice. 7-Ketocholesterol can rapidly diffuse into neuronal cell nuclei causing DNA damage and activation of PARP-1 (Diestel et al., 2003). ROS-induced hyper activation of PARP-1 can result in NADþ and ATP depletion leading to cell death via apoptosis (Bouchard et al., 2003; Burkle, 2005). Neuronal cells are particularly vulnerable to elevated PARP-1 activity, since NADþ is an essential cofactor for oxidative phosphorylation and ATP generation (Arraki et al., 2004). Accordingly PARP inhibitors have been used to prevent cytotoxicity in EAE mice (Farez et al. (2009)). If ROS is the key player that indirectly depletes NADþ, how is ROS generated in MS?
MS is an archetypal inflammatory brain disease associated with the recruitment of activated T-cells and macrophages from the periphery into the neural parenchyma (Lassmann, 2008). These activated immune cells can initiate a number of cytokine-mediated inflammatory responses including the macrophage activating cytokine interferon gamma (IFN-γ). Poor treatment responses with interferon-β have been shown to induce a 4-fold increase in IFN-γ serum mRNA expression (Cucci et al. (2010)). IFN-γ can enhance ROS generation, which can rapidly deplete NADþ levels in many cell types, including neuronal and glial cells, as a result of DNA damage and increased PARP activation (Grant et al., 2000). A significant loss in intracellular NADþ can subsequently lead to cell death, thus supporting the notion that rapid NADþ depletion is detrimental to cell viability.
Mitochondrial dysfunction, which is believed to play a critical role in the pathogenesis of neurodegenerative dis- orders, also represents a major source of ROS (Heales et al., 1999). In progressive MS, axonal damage may be attributed to impaired axonal energy metabolism (Koch et al., 2006). Dysfunction in the axonal mitochondrial electron transport system can enhance ROS formation (Koch et al., 2006). Reduced electron flow through the mitochondrial respiratory chain, particularly through the inhibition of complex I has been reported by Kumleh et al. (2006) showing similar reduction in complex I activity in a Persian cohort with progressive MS (Kumleh et al., 2006). Given the results of the present study, impaired pyridine nucleotide metabolism may be a consequence of impaired mitochondrial respiratory function, and may, at least in part, be attributed to increased NADH: NADþ ratio inducing free radical production. An important observation when examining results of the circulating pyridine nucleotides NADþ and NADH is that MS patients are exposed to energy deficits which may be due to impaired mitochondria function (Dutta et al., 2006; Qi et al., 2006; Witte et al., 2009). Under conditions of acute/chronic ROS and NAD depletion, neurons are exceptionally vulnerable to axonal degeneration which is a typical hallmark of MS (Suh et al., 2007). Moreover, it is well established that MS patients highly vulnerable to inter-current systemic inflammatory or non-inflammatory conditions, particularly during clinical exacerbation of RRMS (Petzold et al., 2004; Rejdak et al., 2007). Therefore, the effect of reduced serum NADþ/NADH ratio on significant CNS and extra-cerebral pathology cannot be overruled.