Molecular mechanisms linking stress and insulin resistance
Habib Yaribeygi et al
EXCLI Journal 2022:317-334. Published January 2022
RONALD PETERS MD – COMMENTARY
Insulin resistance is found in the majority of Americans and is a key metabolic cause of the “diseases of civilization”: obesity, hypertension, type 2 diabetes, high cholesterol, and coronary artery disease. The natural design of the body is to store fat because it is a key nutrient in the production and maintennce of brain cells and cells through the body. Our ancestors ate fat three times a week and modern Americans often eat fat two or three times a day. The first fat storage site is the muscles and when they are full of fat it is difficult for insulin to get glucose into the cell, hence the term “insulin resistance”. Therefore insulin rises in the blood and contribtes to weight gain and the “all- American” diseases mentioned above. In addition to high fat diet, stress also creates insulin resistance through several mechanisms, as shown in this research article. The epidemic of chronic disease in American is based on the parallel epidemic of high fat diet (beef, chicken, dairy and eggs) and chronic stress. People often don’t have time to excercise but they always find time to worry.
Abstract
To date, there is ample evidence to support the strong relationship between stress and insulin resistance. While diabetes mellitus acts as a potent stress inducer, stress may be an upstream event for insulin resistance as well. It is widely recognized that diabetes mellitus is more prevalent among people who have a stressful lifestyle; however, the underlying mechanisms are not well understood. In the current study, we surveyed the scientific literature for possible interactions between stress and insulin resistance and found that stress can impair glucose homeostasis, working through at least six molecular pathways.
Introduction
The global incidence of diabetes mellitus (DM) is growing rapidly (Andes et al., 2019[6]). This chronic disorder adversely affects most metabolic processes and results in diabetes-induced complications and tissue dysfunction (Forbes and Cooper, 2013[34]). DM underlies many disabilities and/or deaths in humans and presents a major challenge for healthcare provider systems throughout the world (ADA, 2018[2]; Sussman et al., 2020[125]). Therefore, many studies have focused upon developing new preventive and/or therapeutic protocols (Kusminski et al., 2016[64], Wong et al., 2017[137], Yaribeygi et al., 2018[141]). Although the exact pathophysiology of DM is not clearly established, some associated risk factors which increase the risk of insulin resistance and DM are well recognized (Kusminski et al., 2016[64]; Zaccardi et al., 2016[145]; Yaribeygi et al., 2021[140]).
Stress is an inevitable part of everyday life and is defined as any state that imposes undesirable conditions on the subjects (Hatef et al., 2016[44]; Patch and Figueredo, 2017[96]). Many local and systemic physiological pathways, such as neural networks and hormonal systems, are stimulated under stressful situations. Stress may therefore have complicated interactions with a myriad of pathophysiologic states including insulin resistance and DM (Wellen and Hotamisligil, 2005[135]; Yaribeygi et al., 2017[142]; Yaribeygi and Sahraei, 2018[143]). Stress is a very broad term, encompassing allostasis, allostatic load, psychological and emotional burdens, physical forces and biochemical changes (Keay and Bandler, 2001[59]; McEwen, 2000[85]). In the current review, we focus our discussion upon chronic emotional and psychological stresses, which are now recognized to be threats to human health, especially in industrial communities. These types of stresses may be induced by many disparate stimuli, such as chronic load noise, air pollution, divorce, dismissal from work, missing child or parents, among others. Although it is well known that minimal or acute stress might be essential in certain circumstances to improve bodily function by working to accelerate nervous processes and enhancing survival (Yaribeygi et al., 2017[142]), strong associations have linked chronic psychological stress with disorders such as cardiovascular disease and metabolic dysfunction (Yaribeygi et al., 2017[142]). In this context, it has been suggested that stress could increase the risk of insulin resistance and lead to a higher incidence of DM (Surwit et al., 1992[124]; Wellen and Hotamisligil, 2005[135]; Li et al., 2013[68]). Since association between stress and insulin resistance has not been extensively studied, the aim of the current review was to present the possible molecular interactions by which stress can impair insulin signaling and induce insulin resistance.
Physiology of stress and stress response
Stress is a common term referred to as any stimulus imposing undesirable conditions on the subject (Yaribeygi and Sahraei, 2018[143]). This can also be described as any external threat emanating from various sources, including physical, chemical, biological, and psychological agents (known as stressors), which tend to disturb the physiological homeostasis of the body (Yaribeygi and Sahraei, 2018[143]). These stressors commonly induce a reaction, known as the stress response, to regain the physiologic balance of the organism and increase its survival (Yaribeygi and Sahraei, 2018[143]). The stress response is mediated via three major pathways: the hypothalamus-pituitary-adrenal (HPA) axis, the renin-angiotensin system (RAS) and the automatic nervous system (ANS). These pathways are further subclassified into the rapid phase (autonomic nervous system) and the slow phase (HPA axis) of the stress response (Yaribeygi et al., 2017[142]; Yaribeygi and Sahraei, 2018[143]). However, these compensatory responses may further exacerbate any pre-existing condition and act as new threats to the body’s homeostasis (Yaribeygi et al., 2017[142]; Yaribeygi and Sahraei, 2018[143]).
The HPA axis is comprised of three endocrine organs, the hypothalamus, pituitary and adrenal glands (Tsigos and Chrousos, 2002[128]; McCormick et al., 2010[82]). The hypothalamus directs adrenocorticotropic hormone (ACTH) release from the anterior pituitary by releasing Corticotropin Releasing Hormone (CRH, or CRF). Arginine vasopressin (AVP or ADH) is also a pivotal hormone, functioning with synergistic effect on the ACTH response (Tsigos and Chrousos, 2002[128]; Carrasco and Van de Kar, 2003[17]; Herman et al., 2003[46]). CRH is subsequently transported via portal vessels to the pituitary, where it binds to specific receptors and initiates the synthesis of a polypeptide known as Pro-opiomelanocortin (POMC), which is the precursor for smaller peptides such as beta endorphins, ACTH and MSH (Sawchenko, 1987[110]; Herman et al., 2003[46]). ACTH then moves via the circulation to the cortical layer of the adrenal glands and, thereby, induces the biosynthesis and release of two forms of adrenocorticosteroids: glucocorticoids and mineralocorticoids (Cullinan et al., 1995[23]). Glucocorticoids are the final effectors of the HPA axis that are directly involved in the stress response, as well as maintaining the basal level of HPA activity and terminating the stress responses through a negative feedback mechanism (Cullinan et al., 1995[23]; Carrasco and Van de Kar, 2003[17]).
The renin-angiotensin system (RAS), which is primarily involved in regulating body fluids and electrolytes, is also involved in the stress response (Boron and Boulpaep, 2012[12]). This system is highly sensitive to changes in plasma concentration, blood pressure and blood volume, and reacts rapidly by releasing angiotensin II (Khanna et al., 2017[60]). Angiotensin II primarily acts as a very potent vasoconstrictor (Khanna et al., 2017[60]). It also induces the release of aldosterone from the adrenal glands and mediates salt homeostasis (mainly sodium and potassium) (McCormick and Bradshaw, 2006[83]). Renin triggers the conversion of angiotensinogen into angiotensin I which then forms angiotensin II in pulmonary epithelial cells. (McCormick and Bradshaw, 2006[83]). Since receptors of angiotensin II (type 1 (AT1) and type 2 (AT2)) are widely distributed in the brain, RAS activation is closely involved in fast elements of stress responses (Carrasco and Van de Kar, 2003[17]; Saavedra et al., 2005[108]).
The autonomic nervous system (ANS) is another system involved in the stress response (Yaribeygi and Sahraei, 2018[143]). This highly reactive network reacts to any stimuli disturbing body fluids, blood pressure or cardiovascular activities through either the sympathetic or parasympathetic nervous system, resulting in chronotropic and/or inotropic effects (Corr et al., 1986[22]). Activation of the ANS also has numerous metabolic outcomes leading to mobilization of substrates (Cacioppo et al., 1998[15]). While the sympathetic system mainly acts via releasing catecholamines from the adrenal glands, the parasympathetic nervous system mostly acts by activation of the ambiguous nucleus and dorsal motor nucleus of vagal nuclei (Kandel et al., 2000[57]) (Table 1(Tab. 1)).
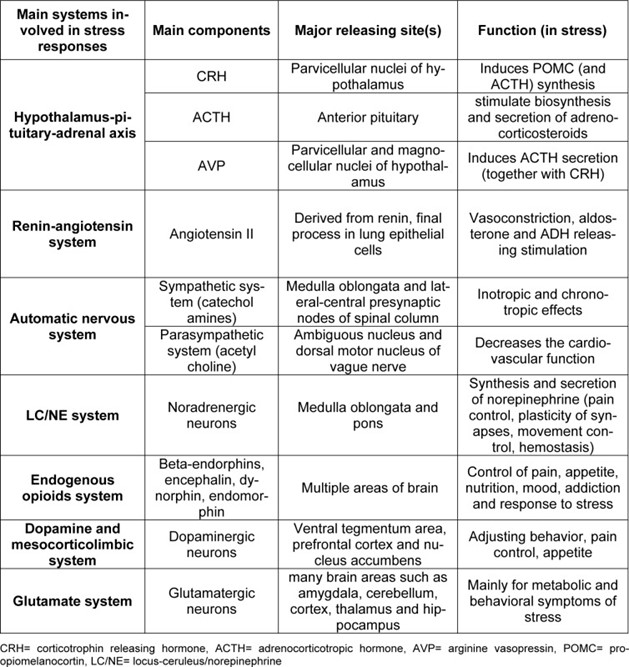
Main physiological systems, and their major components, that are involved in the stress response